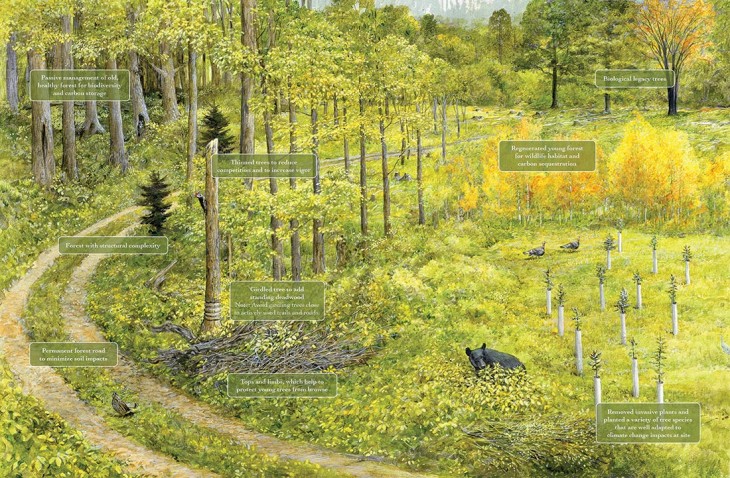
Click here to download a PDF of this article.
Illustrations by Erick Ingraham.
This article is the third in a series focused on forest carbon. Alexandra Kosiba is a forest ecologist, tree physiologist, and assistant professor of forestry at the University of Vermont Extension. She leads the Vermont Forest Carbon Inventory project and provides educational presentations for the Securing Northeast Forest Carbon Program, a federally funded collaboration among state foresters in New England and New York. Click here to learn more about the program, and here to read the first two articles in this series.
Considering the threat of climate change, many landowners and forest managers want to manage forests for the greatest carbon benefit. The question often boils down to: is it better for the climate to manage a forest actively or passively? An active approach involves using silviculture to intentionally alter the composition, structure, and/or growth of the forest and may include removal of trees for personal use or to sell. A passive approach is more hands-off and allows the forest to develop on its own. In a passively managed forest, wood is not harvested.
Passive management has served predominantly as a strategy to protect specific conditions, including sensitive soils, water resources, unique sites, uncommon species, or old-growth forests (footnote 1), or to create wilderness areas. There is growing interest in also using passive management for climate change mitigation. A new term for passive management that includes this carbon focused concern is “proforestation.”
Because active management will reduce the amount of carbon stored in the forest for a period of time following management activities, a passive approach may seem like the best option to keep carbon dioxide out of the atmosphere. However, the decision is not simple. Both active and passive management have carbon benefits and costs, and both are needed to derive the full suite of services we require from forests.
As we seek to make forest management decisions that factor in carbon, it is important to recognize that climate change is a global atmospheric issue. In other words, the atmosphere registers the same warming effect if carbon dioxide is emitted locally or far away. This means that to have a measurable impact on mitigating climate change, we must carefully consider the impacts of our decisions beyond the forest’s edge. We also need to take into account how climate change is stressing forests in new ways, and thereby potentially degrading forests’ capacity to sequester and store carbon.
As landowners weigh these decisions, it is important to recognize that every landowner who chooses to keep their land forested is making a vital contribution to climate change mitigation. Differences between how much carbon could be sequestered or stored by an acre of forest under alternative management approaches are overshadowed by the loss of long-term carbon benefits when that acre is converted to non-forest (footnote 2).
Considering the Carbon Implications of our Resource Needs
To fully understand the outcomes of our management decisions on climate change mitigation, we need to ask: if we don’t use regionally grown wood, what types of resources will we use instead and from where will we get them? How will these decisions affect the amount of carbon dioxide in the atmosphere? Along with keeping forests as forests, a critical strategy for managing forest carbon is to reduce our resource demands for wood and other products. This can involve changing our behavior to consume fewer resources, using wood more efficiently, and/or better utilizing waste products, for example, finding ways to use salvaged wood and wood waste from tree maintenance in towns and cities.
If we continue to use the same amount of wood but stop harvesting timber in our region and instead import wood from elsewhere, the carbon benefits here will be negated because carbon emissions will still occur where the wood is sourced. Plus, there likely will be greater total greenhouse gas emissions from more fossil fuel use due to the longer transportation distances the wood must travel. There also may be other carbon implications that occur when we outsource our wood demands. For example, when we source wood from elsewhere, including parts of the globe with limited environmental regulation, we are potentially funding less sustainable forestry practices, which could lead to environmental degradation in these other places that exacerbate carbon losses (footnote 3). Another concern is that importing wood increases the risk of introducing novel forest insects and diseases to our region, which could increase tree mortality and result in additional carbon losses.
Instead of wood, could we rely on other materials? There are exceptions, but in most cases and for most uses, the alternatives to wood are more carbon-intensive. By using wood instead of other materials such as concrete, steel, fossil fuels, or plastics, we avoid the emissions associated with these materials’ production and transportation – a carbon savings known as a “substitution effect.” In most cases, these other materials are not as easy to reuse, repurpose, or recycle as are wood products.
Managing for Resilient Carbon
Not only is the impact of our resource needs complex, so are the consequences of a rapidly changing climate on forests and the carbon they sequester and store. Some tree species are facing elevated stress because of climate change, and as the frequency and intensity of disturbances increase, there is a higher risk of tree growth reductions and mortality. These stressors could reduce a forest’s carbon sequestration rate or even change a forest from a carbon sink to a carbon source.
Therefore, ensuring the long-term resilience and persistence of forests’ carbon benefits is crucial. To maintain carbon sequestration and storage into the future, a management approach should reflect the specific conditions and potential vulnerabilities of a forest. Active management should foster ecological characteristics that confer resilience, such as diversity, complexity, and redundancy; this is also referred to as “ecological silviculture.” (footnote 4) Importantly, there is no one-size-fits-all tactic; each forest is unique based on its historical land use and management, current characteristics and stressors, future anticipated stressors, larger landscape context, and other goals the landowner may have in addition to carbon.
From a carbon perspective, a passive approach may yield the best outcome for forests with a low risk of carbon loss and a high potential for carbon gain. Passive management may achieve the desired carbon benefits for forests that are diverse, complex, and already functioning well – in other words, forests that have not been significantly altered or degraded by past land use or forest health issues. Passive management for carbon should include monitoring to identify stressors or threats that could harm the health and condition of a forest. If stressors do develop, intervention may be the most effective way to promote the forest’s long-term carbon benefits.
As noted above, a hands-off approach is often a good fit when it aligns with other objectives, such as protecting biodiversity, riparian areas, old-growth or old forests, uncommon species, and unique sites. For example, for a stand of northern white-cedar growing on wet soils, a passive approach may help achieve a landowner’s goals of reducing carbon losses and protecting biodiversity. During active management, use of equipment can compact the sensitive soils and access roads can alter the hydrology. Plus, northern white-cedar forest swamps provide habitat for several rare or uncommon species, such as showy lady’s slippers.
Active management may be more suitable for forests that could benefit from improvements in terms of diversity, structure, wildlife habitat, and ecosystem functioning to ensure long-term carbon sequestration and storage. Because of past land use decisions, heavy deer browse, and the introduction of invasive plants, insects, diseases, and earthworms, some forests in the region lack the suite of characteristics that confer resilience to climate change–related disturbance and stress. Ecologically informed active management can accelerate the development of resilient forest characteristics, such as the presence of large trees, a diversity of species, variation in tree sizes and ages, ample deadwood, and complex structure.
For instance, consider a stand of white pine trees that grew up on an old field and are roughly the same age. Thoughtful active management can help transition this group of pines to a multiage, multi-species stand and in doing so, provide local wood products; help reduce vulnerability to destructive events such as windstorms, insect outbreaks, and drought; and deliver sustained carbon benefits. Without active management, this stand would likely develop complex structure and species diversity over time, but the transition could take decades to centuries, with carbon fluctuations along the way.
Another example of a common forest condition in the Northeast region is a forest stand dominated by American beech trees that are suffering from beech bark disease. In response to the disease, weakened and dying beech trees produce root sprouts in a dense thicket that shades out most other plants. Because these sprouts are clones of the parent tree and attached to the same root system, they will also succumb to the disease. As a result, the stand will go through a pattern of perpetual growth and decline without ever achieving full carbon potential. If carbon is a goal for this stand, shifting composition to other species may be more likely to achieve desired carbon benefits, create more diversity, and help reduce the stand’s vulnerabilities to additional stressors.
Ecologically focused active management can also facilitate a forest’s adaptation to climate change by promoting the establishment of climate-adapted species – species that scientists believe will be better suited to future conditions likely to occur at a given site. For example, in a warmer climate, oaks and hickories may be able to expand their range into new locations within the region; however, these species require gaps in the forest’s canopy to provide adequate and ample sunlight to establish and thrive. Active management can create these growing spaces, which also support a wide range of forest-dwelling wildlife. For instance, many bird species rely on canopy gaps, dense understories, and a variety of plant species to find insects, fruits, and nesting sites, which may not be present in forests with uninterrupted canopies.
It is important to note that passive and active management approaches can be combined on a forest parcel to achieve multiple goals. Forest management strategies should be flexible and adaptable over time, considering the unique characteristics of each forest, objectives of landowners, and importance of mitigating climate change.
Strategies to Manage Forests for Carbon
There are numerous ways to manage forests for carbon. The most critical strategies, which we can all play a role in regardless of whether we own land, are keeping forests as forests and reducing consumption. Within a forest, many of the strategies listed below can be combined and complement adjacent areas reserved for passive management. A diversified and ecologically informed management approach can promote forest resilience to disturbances and stressors, facilitating adaptation to climate change.
Designating reserves to protect certain features, species, or conditions will retain carbon on site. Reserves can range in size from a group of trees to a much larger area, depending on the landowner objectives and condition of the forest.
Reserving large trees as biological legacies protects the substantial amounts of carbon in these trees and contributes to forest complexity. Large trees are often the oldest trees in a stand and as such are a source of locally adapted seed for future generations. Large trees are also more likely than smaller trees to develop cavities that provide essential habitat for wildlife. As these trees die, their branches and trunks become part of the deadwood pool, where they will continue to provide many ecosystem benefits as they decompose. For landowners interested in the carbon and associated benefits of large trees, proper marking and protection of these trees should be included in the forest management plan.
Enhancing the diversity of tree species is likely to improve carbon sequestration and storage over time. Different tree species occupy unique ecological niches, which can allow for more efficient resource use and carbon sequestration. For example, evergreen trees can photosynthesize when deciduous trees are leafless, while deciduous trees can sequester carbon at higher rates during summer. Species diversity also enhances forests’ resilience to disturbances such as insect outbreaks, frost events, and windstorms, and can facilitate adaptation to climate change with the presence or establishment of future climate-adapted tree species. A diverse forest also supports a wider array of wildlife, contributing to ecosystem health.
Increasing both vertical and horizontal structural complexity of the forest has numerous benefits. A complex forest structure is characterized by variation in tree diameters, standing and downed deadwood, multiple canopy layers, and periodic canopy gaps. Such complexity enhances carbon storage and sequestration, improves resilience to disturbances and stressors, and provides habitat for wildlife. For example, some forest birds, such as the eastern wood-pewee and Canada warbler, require a complex forest that includes canopy gaps to forage for insects or to rear young. Old-growth forests often exhibit these characteristics, and ecological silviculture can create old-growth characteristics on an accelerated time scale, emulating natural disturbances and helping to develop multi-aged and structurally diverse forests.
Implementing thinning practices, including removal of unhealthy-looking trees, those prone to breakage, and those with small crowns, can improve the growth and health of remaining trees and enhance carbon sequestration in the long term. Close tree spacing leads to high competition for light and resources, negatively impacting tree health and growth, and increasing tree mortality. Thinning can also be used to promote species diversity, including those species expected to be better adapted to a future climate. Foresters use stocking charts based on forest type, tree spacing, and average tree size to manage stand density. For example, recommended stocking densities for hardwood forests typically range between 60 and 90 square feet of basal area (footnote 5) per acre. This range strikes a balance between stand-level carbon storage, individual tree sequestration, long-term vigor, and regeneration opportunities. However, thinning may not be suitable for especially dense stands, which could result in windthrow. Thinning treatments can benefit both younger and older forests, reducing competition, promoting diversity, alleviating drought pressure, and enhancing resilience to climate change. One effective practice known as crop tree release involves selectively removing neighboring trees that are growing near the desired (crop) trees, which also creates space for a new cohort of trees to establish.
Allowing natural regeneration or planting in areas with low tree density or no trees will increase carbon sequestration and storage. Formerly forested areas that are currently devoid of trees but not utilized for other purposes can be allowed to regrow naturally as forests, although these areas may require periodic interventions to prevent the spread of invasive plants. In cases where forests have poor natural regeneration, tree planting can be employed. Tree planting can also be a good strategy to increase the number of tree species that are well adapted to future climate conditions. Planting trees can be expensive, however, and local nurseries may not offer a wide array of tree species or have sufficient volumes to meet demands. Trees also may need to be protected from animal browse with cages or other deterrents.
Increasing the amount and distribution of deadwood, both standing and downed, transfers carbon from the live tree carbon pool to the deadwood pool. Over time, deadwood will decompose and in doing so, emit carbon dioxide back to the atmosphere, but some carbon will help build the soil carbon pool. Additionally, creating deadwood can allow the remaining living trees to have more space to grow and sequester carbon. Deadwood also provides other important ecosystem benefits such as moisture retention, reduced soil erosion, nutrient cycling, and wildlife habitat. Larger logs are important as they take longer to decompose, thereby storing carbon for a longer duration, and they also provide greater value to wildlife.
To manage deadwood, leave dying trees undisturbed, and if possible, don’t remove fallen trees. To create more deadwood, selectively cut trees and leave them on the ground. Another method is to kill some trees by girdling them, which involves cutting around the trunk of a tree without causing it to fall. Note that any standing dead or dying tree can be a hazard, so avoid girdling trees near roads, trails, and areas where active management is planned within the next five years. During a timber harvest, leave tops and limbs in the woods, without cutting them into smaller pieces or compacting them with equipment. These tops and limbs contribute to the deadwood carbon pool and protect young trees against deer browse.
Extending the length of time between harvests of commercially viable stands allows trees to grow larger and store more carbon. This approach can also yield higher-quality trees suitable for long-lived wood products, such as flooring, furniture, and building materials, which can store carbon for longer than other wood products, such as paper, cardboard, and firewood. Extending rotations can complement some of the other practices in this list to create structural and ecological attributes in younger stands that resemble those found in old-growth forests. For instance, pairing extended rotations with thinning can accelerate the growth of larger trees and foster a range of tree sizes. However, extending rotations carries the risk of losses from storms or other disturbances, so it may not be suitable for all forest conditions. Delaying a timber harvest may not yield the desired carbon benefits if a forest stand is dominated by unhealthy, low-vigor trees, impacted by forest health issues such as insects or diseases, or if it is severely under- or overstocked.
Ensuring successful regeneration is crucial to sustain the forest’s long-term carbon benefits if age diversity in the stand is lacking. One effective method is to create light-filled growing areas for young trees by removing overstory trees. These canopy gaps can range in size from a small space created by removing a single tree to a larger area. Over time, gaps can be expanded to create a mosaic of tree ages and species. In some cases, removing the understory may also be necessary if it lacks tree species diversity or is in poor condition due to heavy deer browse. The size of the canopy gap should be based on the forest type, site characteristics, and desired species – as individual tree species have specific requirements for success. For instance, red oak and white pine do not thrive in shade and therefore need larger canopy gaps. Some tree species benefit from specific conditions on the forest floor; for example, decaying stumps and logs aid in the establishment of hemlock, red spruce, and yellow birch.
Keep in mind that high densities of invasive plants can outcompete tree seedlings, and deer and other herbivore browse can reduce the diversity and abundance of young trees. To address these issues, landowners may want to use strategies such as mechanical and/or herbicide-based invasives removal, promoting deer hunting, or leaving tree tops and limbs from harvested trees to physically protect regeneration. If there is a lot of deer browse pressure, another option is to create fewer, larger gaps that produce more seedlings than deer can consume.
Minimizing damage to trees and soils caused by equipment, vehicles, and foot traffic protects carbon benefits. Soils play a crucial role in supporting biodiversity, nutrient cycling, water retention, and carbon storage, as highlighted in the second article of this series. Therefore, it is important to take extra precautions to reduce soil disturbances when using equipment and vehicles in the woods. Adhering to best management practices (BMPs, footnote 6) is essential in minimizing the impacts of timber harvesting on soil and water resources.
Traditionally, harvesting during winter when the ground is frozen has been the preferred approach to minimize soil impacts. However, due to climate change, the number of days with frozen ground conditions has decreased and become less consistent, necessitating alternatives to minimize impacts throughout the year. One effective strategy is to establish permanent forest access roads, concentrating impacts to specific areas. Timber mats, corduroy, or bridges can provide equipment access while minimizing compaction and damage to wet soils. Soil damage concerns may also influence the type of management activity. For example, stand-wide partial cutting treatments require equipment to traverse larger portions of the forest, which may lead to more extensive soil impacts. By contrast, cutting groups of trees to create canopy gaps can concentrate impacts within limited areas, thereby reducing the overall soil disturbance. Additionally, it is crucial to consider ways to minimize damage to the remaining trees from equipment. Because wounding can allow wood decay fungi to enter trees, damage to trees can negatively affect their health and growth. Careful planning and utilizing deadwood as shielding can protect standing trees from unintentional stem damage during harvesting operations.
Cultivating and harvesting timber that can be utilized in durable, long-lived products promotes long-term carbon storage. Focusing management activities on enhancing the quality and size of trees can result in a higher proportion of harvested timber that is appropriate for beams, boards, and other long-lasting products that store carbon for decades or even centuries. However, one challenge to this strategy is the limited availability of local mills and markets for specific species and sizes of logs. Even if harvested wood can’t be milled into boards or beams, it may still provide carbon benefits by reducing imports of non-wood alternatives. One example is oriented strand board (OSB), which is a type of engineered wood that uses small wood pieces to make large sheets for flooring and sheathing. There are also new and developing ways to use wood for products such as insulation, which has traditionally relied on more carbon-intensive materials. And in the Northeast, many homes rely on wood heat during cold winter months instead of using fossil fuels. Depending on the forest’s condition, harvesting wood can help achieve forest health and resiliency goals while providing financial support for forest management efforts.
How to Manage Forests is a Complex Decision
How to best manage a forest for carbon – including whether to employ active management strategies or to take a hands-off passive approach – requires us to think simultaneously about the specific characteristics and values of the forests in our care, and to consider how management choices interact with larger forces, such as natural resource markets and changing climate conditions. Recognizing that we rely on forests for many services means being considerate and intentional about forest conservation and stewardship, and ensuring the integrity of the larger forested landscape. Thoughtful planning can help us consider where to apply different types of management and importantly, how to keep forests, and all the many benefits they provide, healthy in an uncertain future.
Resources for More Information:
Catanzaro, P and D’Amato, A (2019). “Forest Carbon: An Essential Natural Solution to Climate Change.” University of Vermont and University of Massachusetts Extension.
Marx, L, Zimmerman, C, Ontl, T, and Janowiak, M (2021). “Healthy Forests for our Future: A management guide to increase carbon storage in Northeast forests.” The Nature Conservancy and Northern Institute of Applied Climate Science. Massachusetts DCR. “Caring for Your Woods: Managing for Forest Carbon” (2021).
Palik B, D’Amato A, Franklin J, and Johnson KN. Ecological Silviculture (Waveland Press, 2021).
D’Amato, A and Catanzaro, P (2022). “Restoring Old-Growth Characteristics to New England’s and New York’s Forests.” University of Vermont and University of Massachusetts Extension.
Kosiba, AM (2022). “12 Steps for Climate Resilience: Managing Your Forest with Climate Change in Mind.” Vermont Woodlands Association, Vermont Forests, Parks & Recreation, UVM Extension, and Vermont Tree Farm.
Audubon’s Foresters for the Birds. Available in multiple states.
Footnotes
1. Old-growth forests are forests that were never directly impacted by intensive human land use. See D’Amato, A and Catanzaro, P (2022), Restoring Old-Growth Characteristics to New England’s and New York’s Forests.
2. For more information, see Williams CA, Hasler N, Xi L (2021). “Avoided Deforestation: A Climate Mitigation Opportunity in New England and New York.” Report for United States Climate Alliance Natural and Working Lands Research Program.
3. There are additional social and economic consequences of outsourcing our resource demands.
4. For more information, see Palik B, D’Amato A, Franklin J, and Johnson KN. Ecological Silviculture (Waveland Press, 2021).
5. Basal area is a way to describe the density of trees in a forest. It is measured by summing the cross-sectional area of each tree’s trunk measured at breast height (4½ feet above ground).
6. BMPs are guidelines released by state agencies that provide recommendations for sustainable forest management. In Vermont, these guidelines are called Acceptable Management Practices (AMPs).