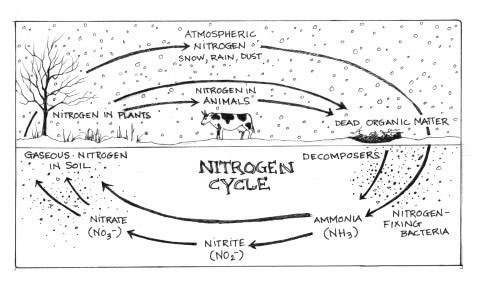
Our lawn is a scruffy assortment of grasses and weeds that receives zero care apart from mowing. The beleaguered grass struggles in the face of competition. However, green grass persists in areas intermingled with clover.
This casual observation has a basis in fact. Clover is a nitrogen-fixing legume, nature’s equivalent to the synthetic nitrogen fertilizer that’s available in the lawn-care section at your local feed store. As the clover dies and breaks down, the grasses around it are able to reap a nitrogen windfall.
Nitrogen is a funny element. It’s everywhere (air is 78 percent nitrogen), and it’s crucial to plant and animal life. Yet most living things have no way of using it – nitrogen atoms are paired by triple bonds that are extremely hard to break apart and convert, or ‘fix’, into chemicals useful to living organisms.
In prehistoric times, nitrogen was fixed in one of two ways: either the intense heat of lighting broke apart the paired nitrogen atoms, allowing combination with hydrogen to make ammonia, or, less dramatically (if more effectively), microbes and plants worked cooperatively to pull it out of the air.
Here’s where clover comes in. Clover and other legumes draw specialized bacteria into their roots where they are housed in nodules that look like little swollen balls. The bacteria are able to convert atmospheric nitrogen into a form that that the plant can use. Meanwhile, the bacteria benefit by feeding on the plant’s sugars.
Although legumes are considered the classical nitrogen-fixing plants, they are not alone in being able to do so. Certain grasses, notably tropical rice and sugarcane and temperate rye and buckwheat, also host nitrogen-fixing bacteria, though they need a little previously-fixed nitrogen to jump-start the process.
Nitrogen-fixers eventually die and decompose, leaving behind organic matter and simple nitrogen compounds, including nitrates that are absorbed by other plants. Animals eat these plants and convert the plant’s nitrogen to animal tissues. All plants and animals ultimately die, decompose, and release their organic matter and nitrogen compounds to the soil. This is the front side of the nitrogen cycle.
The back side of the nitrogen cycle, called de-nitrification, occurs when nitrogen returns to the atmosphere. Specialized bacteria break down organic matter that contains nitrogen, releasing nitrogen gas in the process. De-nitrification happens most commonly in saturated soil, particularly wetlands and aquatic environments.
In prehistoric times, nitrogen fixation and de-nitrification were roughly in balance. Enter humans, who increased nitrogen fixation by cultivating more legumes and grasses to boost soil fertility. Then in 1909, things took a dramatic turn. A German chemist named Fritz Haber made a profound breakthrough - a way to synthetically fix nitrogen. By heating methane plus nitrogen gas under high pressure, he created ammonia that could be converted to nitrate fertilizer. Prior to this revolution, agricultural nitrogen fixation stood at about 16 million tons of nitrogen per year. Today about 100 million tons per year of nitrogen in fertilizer are used on crops globally.
Haber’s process is not the only turbo-charging of the nitrogen cycle. When fossil fuels are burned in power plants and automobile engines, nitrogen and oxygen from air react together, producing nitrogen oxides. In 2000, about 27 million tons of nitrogen were fixed in this way.
So what is the upshot of all this extra nitrogen fixation? One consequence is that the world grows more crops and supports more humans. Astoundingly, though, only 12 percent of the nitrogen added to crops ends up being consumed by people. The rest remains in various forms in the environment, with about 2.5 percent staying in the soil as increased organic matter and 80 percent running off the land as excess fertilizer and animal waste.
Wetlands and rivers de-nitrify some runoff, returning the element to the atmospheric pool, as do anaerobic manure lagoons. But there’s only so much these systems can handle, and, even so, about half the wetlands in the United States have already been drained for other uses. The rest of the nitrogen-rich runoff reaches the ocean and fuels the growth of enormous algae populations. Decaying algae deplete oxygen, decimating marine life over hundreds of square miles. The east coast of the U.S. is dotted with dead zones, and worldwide, the number of large dead zones has increased from 49 in the 1960s to 405 in 2008.
The impact on the natural world of excessive fixed nitrogen is still being studied. Many ecosystems will not show the full effects for decades or even hundreds of years. In the meantime, we need some sort of discovery equal to Haber’s that will accelerate the de-nitrification side of the equation and bring the nitrogen cycle back into balance.